Imagine seeing a shooting star streak across the night sky as it burns bright in the atmosphere. How would you feel? Maybe lucky that you had seen a relatively rare event or maybe awe and excitement at witnessing a visitor from space. For members of the Global Fireball Observatory however, you’d feel hope that a rock dropped by that shooting star will reveal insights about our origins in space and the dangers these rocks pose to the Earth.
The Global Fireball Observatory is an international collaboration of sky-watchers established in 2017, bringing together over 19 institutions within Australia, USA, Canada, Morocco, Saudi Arabia, UK, and Argentina. Its aim is to set up fireball observatories across the world to maximise the area of coverage, tracking where these fireballs come from and locating whatever meteorites drop onto land.
A paper1 published in October in Planetary and Space Science describes how the Global Fireball Observatory operates to help scientists discover the origins of the Solar System and build our knowledge about the potential for devastating impacts on Earth of any large rocks falling from space.
Dr Hadrien Devillepoix of Curtin University’s Space Science and Technology Centre was co-author of the paper and chatted to Lab Down Under about the how the Global Fireball Observatory can assist humanity and where the idea came from.
Extraterrestrial flight paths to our origins
Data collected from the Global Fireball Observatory can help scientists discover where these fireballs come from in the Solar System. By uncovering the makeup and composition of these rocks and considering this along with their place of origin, it is possible to determine how they formed, which gives us clues about the birth and formation of the Solar System itself.
Through long-exposure images, the GFO team captures the trajectories of these objects as they blaze across the sky, and are able to extend this path both backwards and forwards to determine where the rock originated from in the Solar System and where it ultimately hit on Earth. Most meteorites that have been discovered originated in the main asteroid belt, a massive ring of rocks orbiting the Sun that lies between Mars and Jupiter.
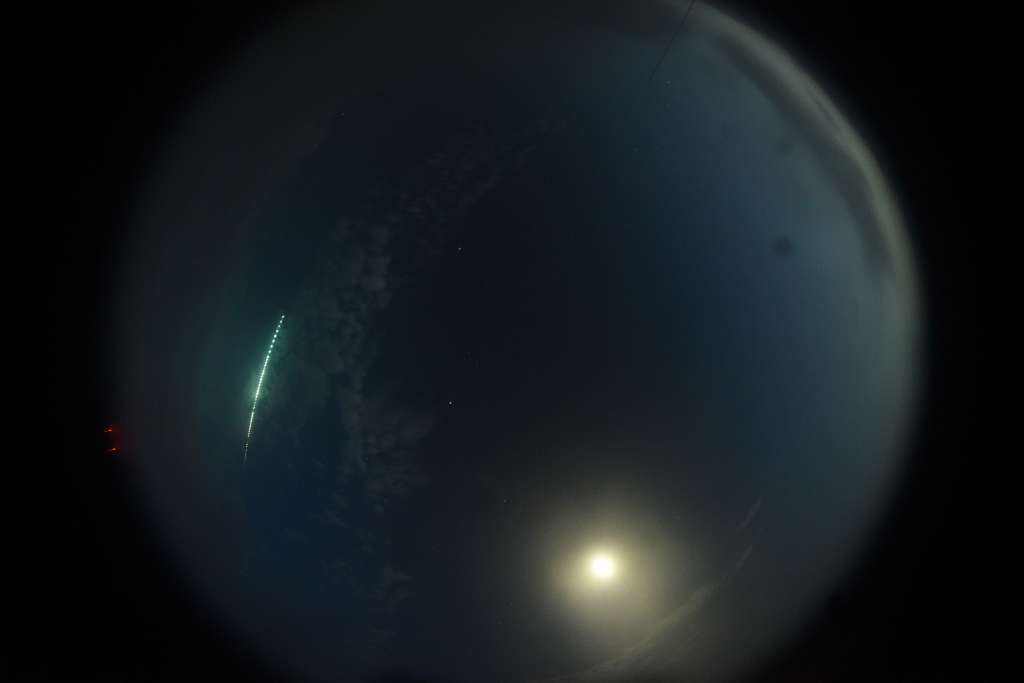
Image: Fireball from the Forrest DFN site, Nullarbor Plain, Western Australia. Picture by DFN Curtin. Used with permission.
Meteors coming from the asteroid belt typically hit the Earth’s atmosphere at around 10 to 20 kilometres per second which, while admittedly fast, is slow enough that some of them do not completely burn up on entry. By determining where these rocks come from and analysing the specimens we recover, scientists can learn a lot about how they and the Solar System formed, Dr Devillepoix said.
“Some asteroids we think are just the remnants of cores of baby planets. They’re usually made up of metal, so that’s how we could get some iron meteorites. Iron meteorites are quite rare, so we’re really looking forwards to get one of those.”
Other meteorites could come from Mars or the Moon, being thrown up by other impacts, but these are quite rare and have yet to be spotted by the Global Fireball Observatory on Earth, Dr Devillepoix said.
The GFO is more economical than going into space to retrieve samples from asteroids, the Moon or Mars as well, he added, because by simply waiting, these specimens could fall from space, allowing us to collect them and analyse them without leaving the Earth.
Keeping our eyes on danger from above
Another use stemming from the Global Fireball Observatory is its ability to track potential sources of dangerous rocks which could impact Earth, resulting in millions of dollars worth of damage as well as injury and death.
Dr Devillepoix referred back to the Chelyabinsk meteor which impacted Russia in 2013 and was made famous by countless pieces of dashcam footage. The impact injured 1,500 people and caused US$33 million worth of damage.
In the scheme of things, the Chelyabinsk meteor was not massive, being only 10 times larger than the fireballs that the Global Fireball Observatory witnesses on a regular basis, Dr Devillepoix said. However, even observing the smaller ones was important in case we stumble upon a stream of them, he added.
“If we do find a stream of them, well we might be wondering whether there was a 10 or even a 100 metre object lying in this stream and whether we should be worried about it. So that’s one thing the GFO tells us about.”
Finally, the network’s observations may also assist with any plans to mine asteroids in the future. One of the Global Fireball Observatory’s prior research papers2, conducted by PhD student Patrick Shober, discovered a meteorite that had actually orbited the Earth like a “mini-Moon” before it entered our atmosphere, Dr Devillepoix said.
“Those objects are really interesting from an asteroid mining point of view. Asteroids typically have lots of metals in them and all the metal hasn’t sunk to the core. So if you wanted to mine an asteroid, one that’s already orbiting the Earth would be a lot easier than going after it somewhere in the Solar System.”
Capturing visitors from outer space
The idea of using cameras to spot meteorites was nothing new, Dr Devillepoix said, with the first photo of a meteor being taken by Austro-Hungarian astronomer Ladislaus Weinek in November 1885. In the 1950s, scientists in Czechoslovakia started putting cameras out to try and track these bright fireballs that streaked across the sky.
The Czech scientists recorded their first meteorite in 1959 and tracked its trajectory back to the main asteroid belt. Off the back of the Czech researchers’ success, observatories were built in the United States and Canada. However, they did not actually recover too many meteorites because the rocks themselves were particularly hard to find.
“So meteorites, as they burn up through the atmosphere, develop this fusion crust around them which is black. Finding a black thing in a dark background whether it’s a forest or a field is pretty difficult, so that was a major problem for recovering those rocks,” Dr Devillepoix said.
This was how Australia entered the picture and the Curtin University-based Desert Fireball Network was created in the mid-2000s, he continued, because it was much easier to find these black meteorites on the lighter coloured soil of places like the Nullarbor Plain.
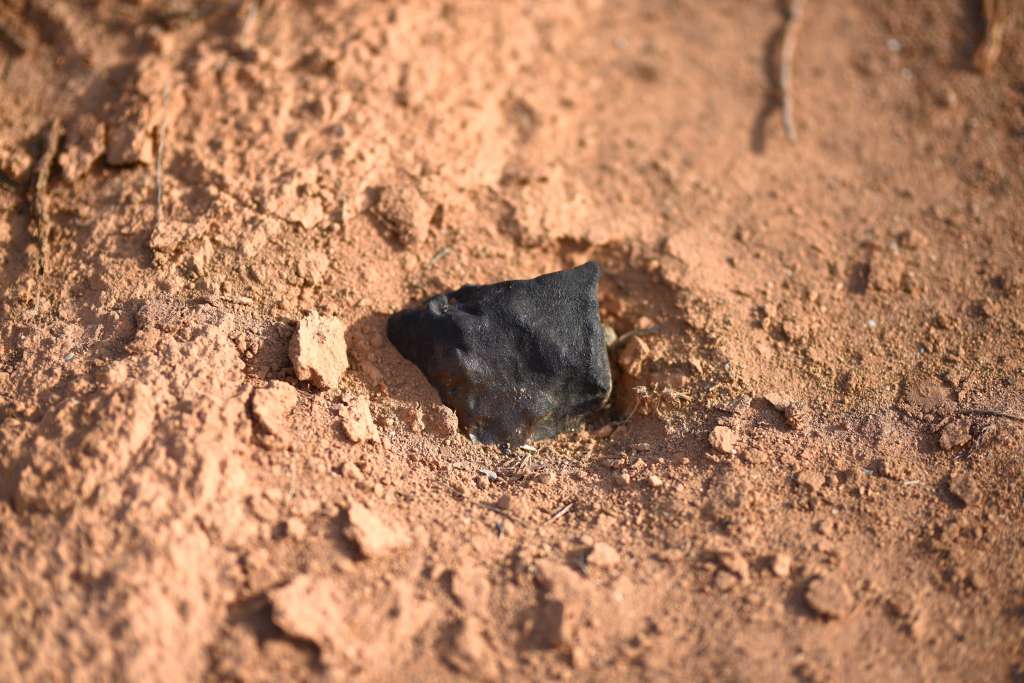
Image: Meteorite recovered from Madura DFN site, Nullarbor Plain, Western Australia. Picture by Dr Hadrien Devillepoix. Used with permission.
Once the DFN was established, it quickly became an excellent proof-of-concept, Dr Devillepoix said, finding its first meteorite which fell onto Lake Eyre in South Australia in 2015. Using the images, scientists were able to calculate where the rock fell to within a reasonable accuracy, allowing them to locate and retrieve the specimen.
On the back of this, the Global Fireball Observatory was born, with new sites established with the help of 19 universities and research groups located worldwide. While some of the sites, such as those in Saudi Arabia, Oman and Morocco, are in desert type environments, others like those in Canada, California and the United Kingdom are not.
A custom setup to track a fireball’s flight
Back when it was first created, the Desert Fireball Network used film cameras because the digital cameras of the time were not of sufficiently high resolution. Night-long exposures took images of the fireballs moving across the heavens and a home-built mechanism would change the film automatically. Someone would then drive out and collect the film once a month and send it to the Czech Republic for developing.
“Within two years, the first meteorite was recovered from that network and another one a couple of years later in 2010. So it was a really successful trial. Because of the size and mechanical complexity of the systems, the network was really hard to operate. The cameras were on the order of $150,000 each,” Dr Devillepoix said.
Once high resolution digital cameras became cheaper, the DFN upgraded their systems. These days, each observatory consists of a Nikon D810 DSLR camera with a fish-eye lens attached to take pictures of the entire sky. Fireballs, each of which last only a few seconds, are captured in 30-second long exposures with a liquid crystal shutter opening and closing to help determine the fireball’s speed.
“You can imagine if you’re just taking a long exposure of the fireball, it’s just a very long streak. But you don’t know which position corresponds to which time, so you can’t calculate velocity which is quite important. So what we do is we have a little liquid crystal shutter in between the lens and the sensor that flicks on and off. This basically breaks the fireball trajectory down so we can figure out the speed,” Dr Devillepoix told Lab Down Under.
Attached to the camera is a PC, some large hard drives to store the data, and a custom printed circuit board (PCD) and micro-controller. The latter two components help turn the components on and off to conserve power coming in from the attached solar panels and battery.
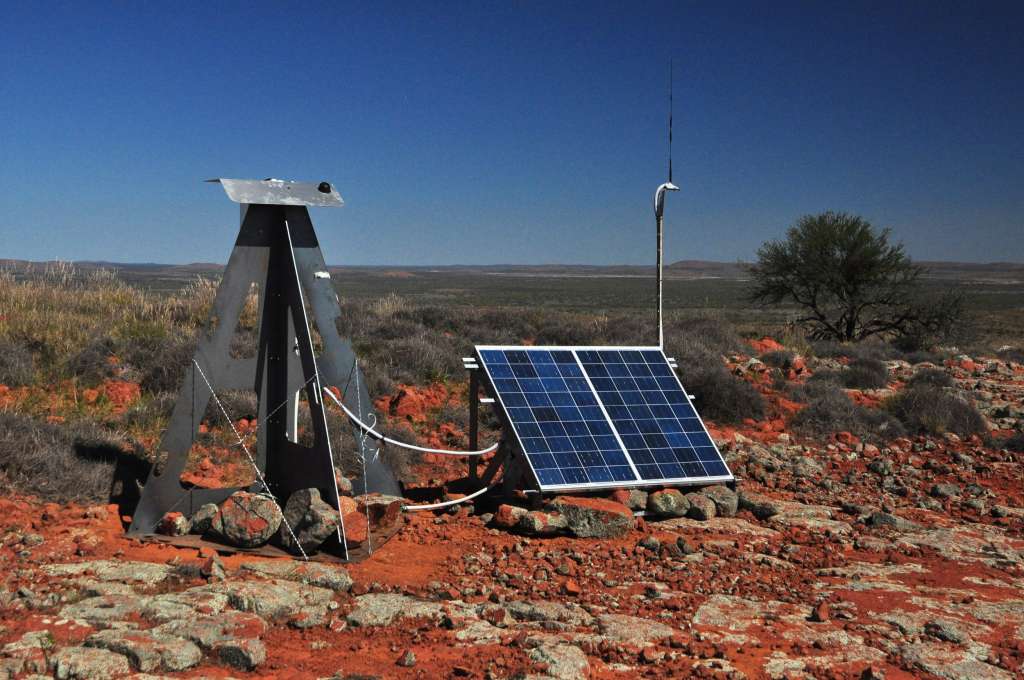
Image: DFN observatory setup (camera on left, solar panels and processing unit on right). Picture by Desert Fireball Network. Used with permission.
The entire setup was expected to last for years out in the desert dust and heat, Dr Devillepoix said, with the Nikon cameras excelling in their task. While the specs for the Nikon D810 claim the shutters can take 200,000 pictures, those used in the DFN far exceeded that number with some taking one million photos.
“I think the longest running we have is still clicking is getting close to two million pictures. So it’s quite incredible when you think about all those mechanical parts — you’ve got the mirror, you’ve got the shutter and all that stuff. Two million clicks and it’s still going,” he told Lab Down Under.
Narrowing down the search area
By measuring the trajectory of the fireball across each image, members of the Global Fireball Observatory can track where each meteorite has fallen within a certain square kilometre area. This is because during the last part of the rock’s flight — called the dark phase — it is no longer burning up and has decelerated enough that it can be buffeted around by the wind.
“How it’s affected by the winds highly depends on its mass, shape, and density. That is quite hard to observe during the bright flight phase. The unknowns around its properties mean there’s quite a bit of uncertainty of how much it’s being pushed by the wind. So you usually end up with what we call a ‘fall line’ that can be a couple of kilometres long, sometimes longer in bad cases,” Dr Devillepoix said.
Sometimes though, it’s possible to just stumble upon these meteorites with Dr Devillepoix almost tripping over one going back to his car in July this year. Travelling out to the Nullarbor to collect more data on the meteorite, which fell in November last year, the trip was meant to prepare a search team which would be going out to retrieve the specimen later on.
“We did a very short trip, five days around the Nullarbor. We quickly checked the fall area where we calculated it might have fallen and we basically found it just after a couple of minutes of searching. We were just looking at the vegetation on the ground just to know what our colleagues were walking into two weeks later and as we were walking back to the car we essentially walked on it. So we were very lucky on that one,” he said.
Tapping the power of teamwork
A collaboration such as the Global Fireball Observatory was important because it standardised the equipment, observation procedures and processing methods across all observation sites worldwide, Dr Devillepoix said.
“If everybody used a camera and lens which they had first developed themselves, they would spend a lot of time creating that. Also writing the software routines to process that data can be quite costly if you have to work with everybody’s camera,” he told Lab down Under.
“Whereas if you’ve got smaller range of equipment across the world, it makes it a lot easier. All the data reduction for the GFO is done here in Perth, mostly automatically by algorithms, and that’s facilitated by the fact that it’s all coming from very similar looking cameras and lenses.”
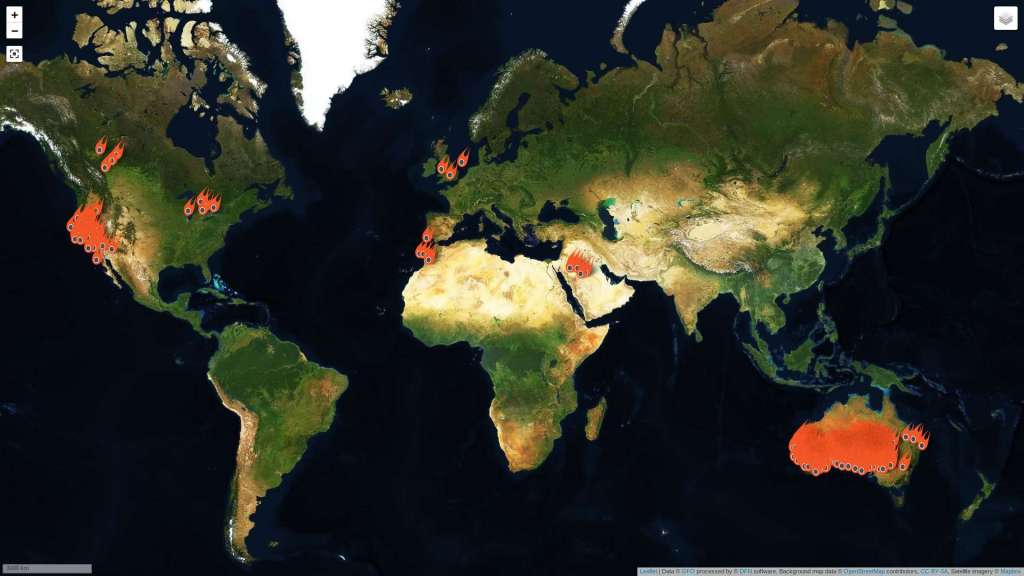
Image: GFO Fireball Detections as of 23/07/2020. Picture by Dr Hadrien Devillepoix. Used with permission.
Another benefit is that a global collaboration gives access to a wider range of experts than that available just here in Australia, Dr Devillepoix said.
“Even if we find a meteorite here, we don’t have all the expertise or all the machines to do the analysis of those rocks. We actually send them out to others for analysis and that’s how we learn about them, how old they are, how they’ve formed, what’s in them, and all of that. So collaboration from this point of view is really important.”
Plans to catch more space Pokémon
One aim of the Global Fireball Observatory is to cover two per cent of the Earth’s surface. With meteorite-dropping fireballs quite rare, the wider the surface area covered by these cameras, the higher the chances of actually spotting them and retrieving them.
“The reason we want to get as many meteorites as possible is because there are lots of different types of them. It’s not because we just like putting them on our shelves and showing them off. There are so many different types that statistically we have to observe a lot to collect them all. It’s sort of like Pokémon when you think about it,” Dr Devillepoix said.
Further work on the GFO will also involve making the process of searching for and locating meteorites more efficient. Currently, a team of six people has to go and walk up and down the search area for two weeks in order to locate these fallen rocks.
One idea involves using drones to survey the area initially and adding machine learning to teach the algorithm how to actually spot a meteorite from the photos taken. This has been the work of PhD student Seamus Anderson of Curtin University, who recently published an article detailing the technique3.
“So we actually haven’t found one with that technique yet but after the last two meteorites we found, before we actually touched them, we took pictures of those rocks on the ground, ran that through the algorithm, and the algorithm in both cases said it was a meteorite. So if we’d actually done our surveying using the drone, we would have found them,” Dr Devillepoix said.
If successfully implemented in practice, this technique will save a lot of time searching for meteorites and allow the GFO team to visit a wider variety of sites to locate even more specimens.
Other improvements such as using larger, fixed wing drones to survey broader search areas and avoid the need to physically visit more remote sites were also possible, Dr Devillepoix said.
“I mean there are lots of crazy ways you could think about how you could make this more efficient, more automated. But I think just not having to walk up and down with humans for two weeks is going to be a big step up even if we still have to drive there.”
As well as Curtin University, the Australian institutes involved in the GFO are University of Sydney, Australian National University, University of Southern Queensland, Monash University and Macquarie University.
Author’s note: If you enjoyed this article, you can follow Lab Down Under on Facebook and LinkedIn or support me on Patreon. I also have my own personal Twitter account where I’ll be sharing my latest stories and any other items of interest. Finally, you can subscribe here to get my weekly blogs in your inbox.
1 Devillepoix H, Cupák M, Bland P, Sansom E, Towner M, Howie R, Hartig B, Jansen-Sturgeon T, Shober P, Anderson S, Benedix G, Busan D, Sayers R, Jenniskens P, Albers J, Herd C, Hill P, Brown P, Krzeminski Z, Osinski G, Chennaoui Aoudjehane H, Benkhaldoun Z, Jabiri A, Guennoun M, Barka A, Darhmaoui H, Daly L, Collins G, McMullan S, Suttle M, Ireland T, Bonning G, Baeza L, Alrefay T, Horner J, Swindle T, Hergenrother C, Fries M, Tomkins A, Langendam A, Rushmer T, O’Neill C, Janches D, Hormaechea J, Shaw C, Young J, Alexander M, Mardon A, Tate J. A Global Fireball Observatory. Planetary and Space Science 191 (2020) 105036.
2 Shober P, Jansen-Sturgeon T, Sansom E, Devillepoix H, Bland P, Cupák M, Towner M, Howie R, Hartig B. Identification of a Minimoon Fireball. The Astronomical Journal, October 2019, 158, 183.
3 Anderson A, Towner M, Bland P, Haikings C, Volante W, Sansom E, Devillepoix H, Shober P, Hartig B, Cupak M, Jansen-Sturgeon T, Howie R, Benedix G, Deacon G. Machine Learning for Semi-Automated Meteorite Recovery. Accepted for publication in Meteoritics & Planetary Science.
Featured image: Gray buildings and river, digital art, landscape, night, stars HD wallpaper. Picture from Wallpaper Flare.